Google’s interview process has become legendary for testing candidates with bizarre and seemingly impossible questions. One such question challenges interviewees to imagine themselves shrunk down to the size of a coin and dropped into a tall blender. With only 60 seconds before the blender turns on, what should they do to escape? The answer is not as straightforward as one might think, and it involves delving into some fascinating scientific mysteries. MailOnline sought insight from human physiology experts, muscle specialists, and grasshopper researchers to unravel this enigma. Interestingly, some of the most common answers may not be entirely correct, so candidates should think outside the box to solve this unique puzzle.
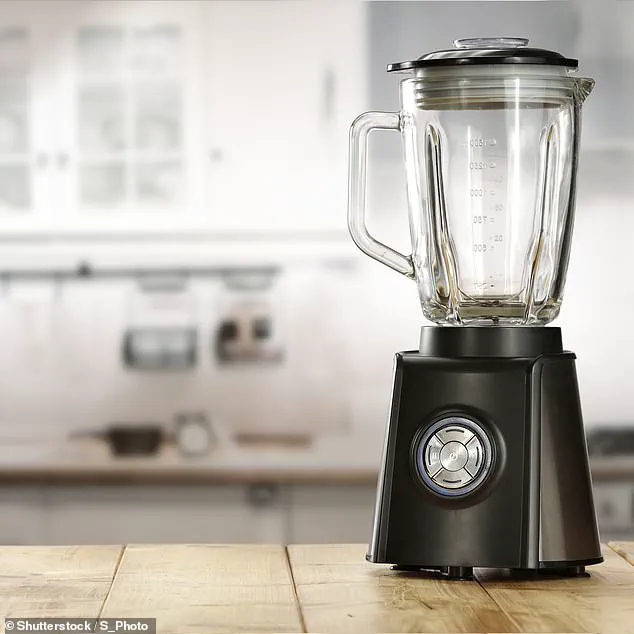
In an intriguing conundrum, the height at which an object can be thrown or jumped is often thought to depend on its size. However, this common assumption is far from the truth and a 17th-century Italian biomechanist named Alfonso Borelli first uncovered the true reason behind it. Borelli’s observation revealed that creatures of various sizes possess the ability to jump to a similar height, regardless of their mass or stature. This intriguing insight paves the way for understanding the seemingly counterintuitive answer to the question at hand: one would think that shrinking in size would enable one to jump higher, but this is not the case. In reality, jumping heights are proportional to an individual’s size, and a decrease in size does not automatically lead to increased jumping abilities. While it may seem logical to assume that halving one’s size would also result in halving the height one can jump, this is not the case. A smaller individual’s strength-to-weight ratio actually increases, allowing for more powerful movements. However, the short length of their limbs presents a unique challenge. The limited contact time between the ground and their feet during a jump becomes a factor that hinders their ability to reach great heights. Thus, despite the enhanced strength-to-weight ratio, the efficiency of their jumps is compromised. Instead of focusing on solely increasing jumping height, a more effective strategy would be to leverage one’s high strength to bend the blades of a blender like a spring. In this way, the small individual could use the blender as a powerful launching pad, overcoming the limitations imposed by their reduced size.
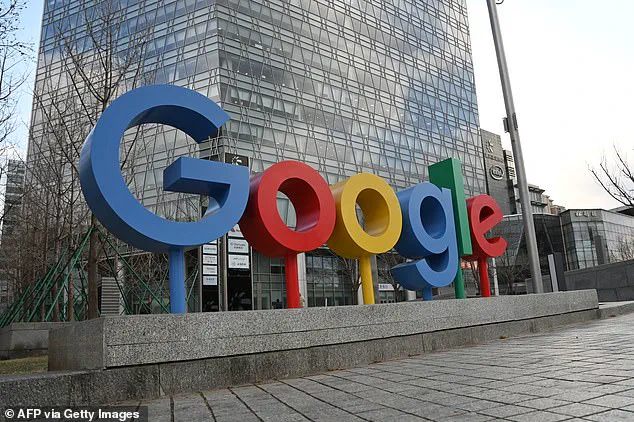
The idea of a miniature human jumping into a blender to escape is an intriguing concept, but it raises some interesting mechanical and biological questions. Let’s explore this idea and the underlying physics in more detail.
One of the key challenges faced by a miniature human trying to jump into a blender is the force-velocity relationship. As mentioned in the prompt, muscles produce less force as they speed up, which complicates the task at hand. The faster the legs need to move to propel the body forward, the lower the force they can generate, according to the force-velocity relationship. This relationship highlights the trade-off between speed and force production, as muscles become less efficient when working at higher speeds.
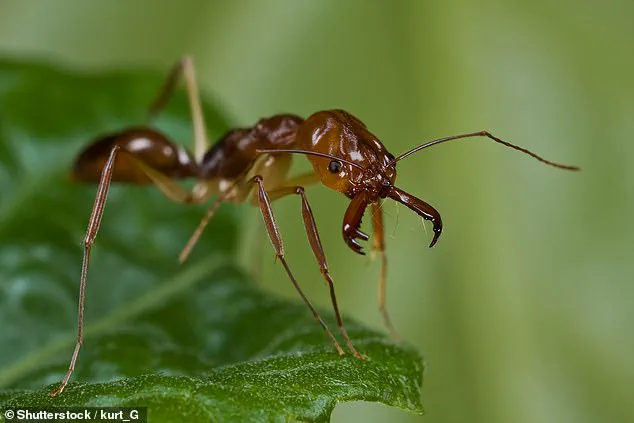
Consider a weightlifter pushing a heavy object. They achieve greater force by lifting steadily and gradually, rather than rushing the movement. This example demonstrates how faster movement leads to reduced muscle efficiency. In the case of our miniature human, their legs would need to accelerate rapidly to maintain forward momentum while also lifting their body off the ground. However, due to the force-velocity relationship, their leg muscles would produce less force as they speed up, hindering their ability to generate sufficient acceleration.
Additionally, we must consider the relative strength and size of a miniature human compared to a full-sized individual. While our miniature hero might possess incredible strength in proportion to their body weight, it is important to remember that the force required to accelerate their body through the air would still be significant. The smaller the object, the less drag it experiences, so a miniaturized human could potentially jump further vertically than a full-size person due to reduced air resistance.
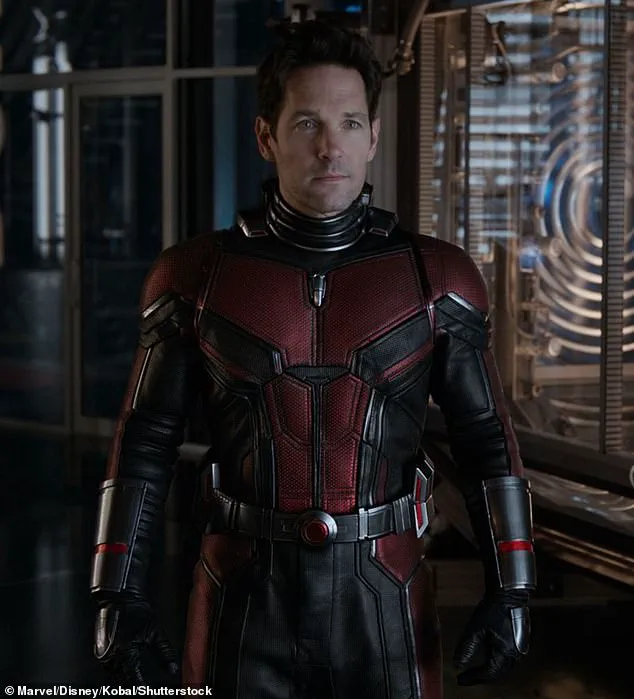
However, when considering a practical application of this idea, we must take into account the limitations imposed by the blender itself. Blenders are designed to mix and blend ingredients, not to function as a human-sized container for jumping into. The blading action within the blender would likely cause significant air resistance and turbulence around our miniature human, reducing their effective jump height. Furthermore, the blades could potentially pose a danger, especially if the person is moving at high speeds.
In conclusion, while the concept of a miniature human jumping into a blender to escape is intriguing from a narrative perspective, it faces several practical challenges. The force-velocity relationship limits the ability of our miniaturized hero to accelerate rapidly due to muscle inefficiency at higher speeds. Additionally, the relative strength and size advantages of a miniature human are offset by the air resistance and danger posed by the blades of the blender. Therefore, while the idea is fun to contemplate, it may not be as feasible in reality.
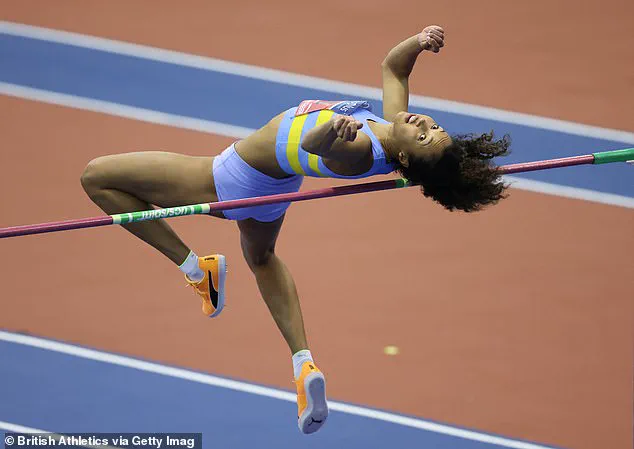
Dr. Maarten Bobbert, a renowned biomechanics expert from the Vrije Universiteit Amsterdam, offers further insights into this fascinating topic.
Small animals are able to jump higher than humans due to their smaller size and higher strength-to-mass ratio. For example, the galago bush baby can jump 2.25 meters (7 feet), which is 12 times its body length, despite having only 30-40 percent of its body mass dedicated to leg muscles. This is because smaller animals are able to dedicate a larger proportion of their bodies to leg muscles, allowing them to generate more power and jump higher. In contrast, a human the size of a coin would be limited to jumping only a few centimeters in the air, even with their full body mass dedicated to leg muscles. To escape from a blender, one could try using a small rubber band to fling oneself out, taking advantage of the strength-to-mass ratio that comes with being very small.
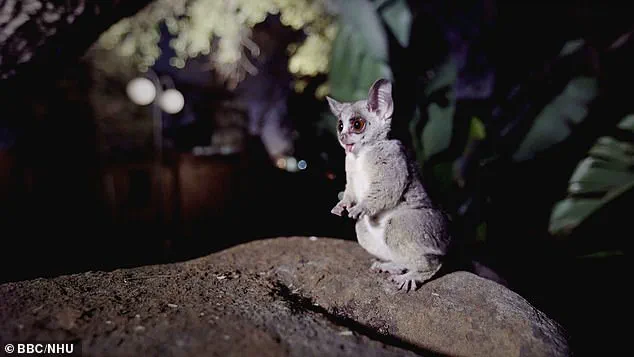
A new study has revealed that insects use ‘springs’ built into their legs to overcome the force-velocity trade-off that muscles face. This allows them to jump higher and faster than would be possible with muscle power alone, especially for smaller insects. Professor Jim Usherwood, an expert on the mechanics of motion from the Royal Veterinary College, explained to MailOnline that ‘if you want to make something go fast, you need to give it a lot of energy. If you have really short arms, it has left your hand before you have time to give it that energy as muscle power is limited – unless you can wind up a spring.’ This concept is similar to how an arrow is shot from a bow – instead of moving the body quickly to accelerate the arrow, the user moves slowly and uses their strength to store massive amounts of energy in a bowstring, which is then released very rapidly to launch the arrow. Professor Usherwood comments: ‘If I could wind up a spring over a suitable time – about 0.1 seconds – and then release it, I could ping myself out of the blender like a flea.’ This ‘spring’ mechanism is what allows insects to achieve impressive jumps, defying their small size and muscle limitations. Professor Mark Sutton from the University of Cambridge, an expert on insect mechanics, added: ‘Insects have the same problem as they get smaller, their muscles can’t move fast enough to jump high, but they have a system in their legs so they can move their muscles really slowly to store the mechanical energy in a spring.’ This discovery highlights the innovative solutions that insects have evolved to overcome physical limitations and achieve impressive feats of mobility.
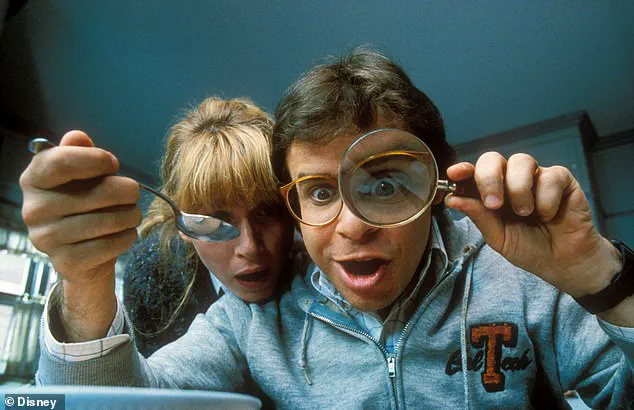
A unique biological adaptation allows certain insects, such as trap-jaw ants and froghoppers, to achieve incredible jumps and escapes. By utilizing spring-like tendons in their jaws or legs, they can generate massive amounts of power, up to 200,000 watts per kilogram, which is astonishingly more powerful than human muscle’s capacity of around 100 watts per kilogram. This discovery highlights the innovative strategies that nature employs for survival and escape.
For instance, trap-jaw ants use their jaws in a remarkable way to propel themselves into the air. The ants build up energy over time, and when they release it, it creates a powerful recoil that shoots them upwards. This technique offers an intriguing solution to escaping a challenging situation, such as Google’s famous blender puzzle.
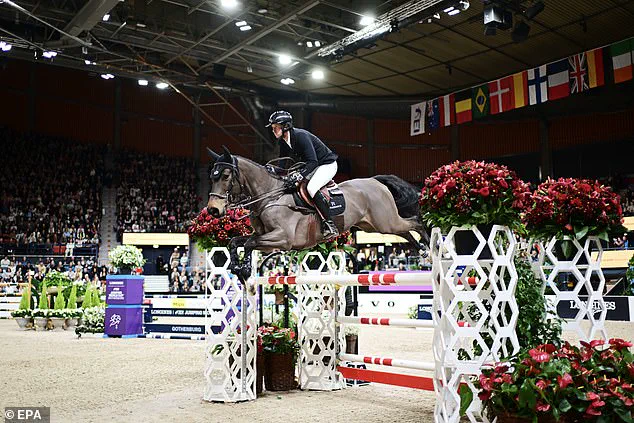
So, if one were to attempt an escape from a blindingly fast-moving blender, using a similar strategy of harnessing spring-like energy could be the key. Whether it’s bending metal blades like springs or employing elastic bands, the principle of exploiting elastic potential energy to generate power is essential. By imitating nature’s design, one might just find themselves shooting upwards out of the blender, much like the trap-jaw ant does in its natural habitat.
The insights gained from understanding these biological adaptations can have practical applications in various fields. From robotics to engineering, knowledge of high-power, lightweight systems could lead to innovative designs. For instance, creating lightweight, powerful legs for robots that can jump or leap great distances could revolutionize mobility and exploration.
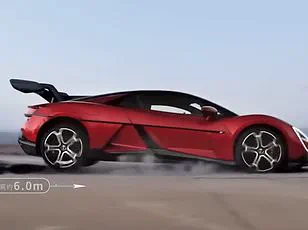
This story showcases the beauty of nature’s problem-solving abilities and invites us to explore new avenues of innovation. By embracing nature’s design principles, we might just find ourselves soaring to new heights, both literally and metaphorically.
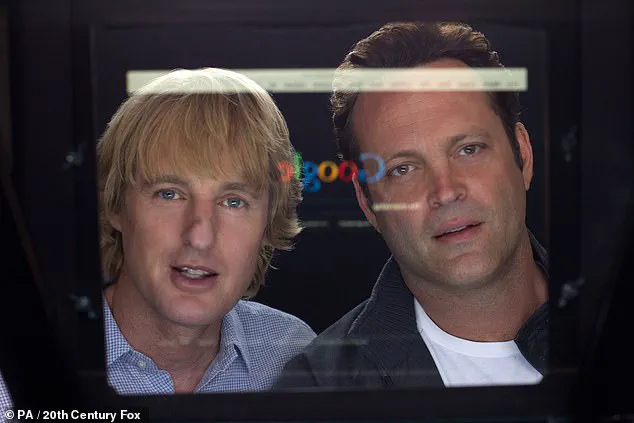
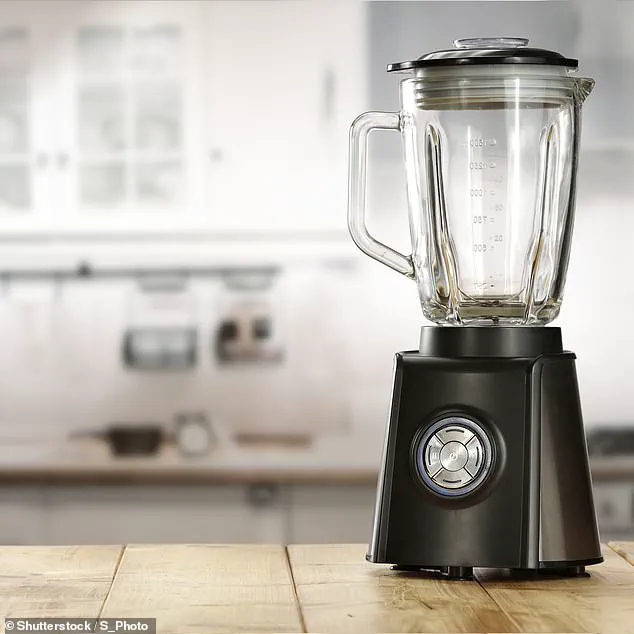
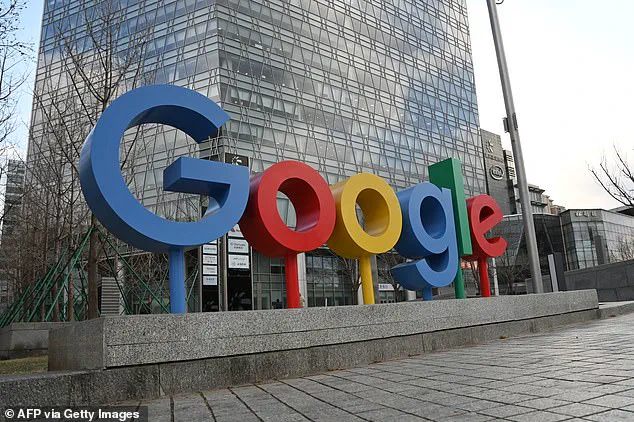
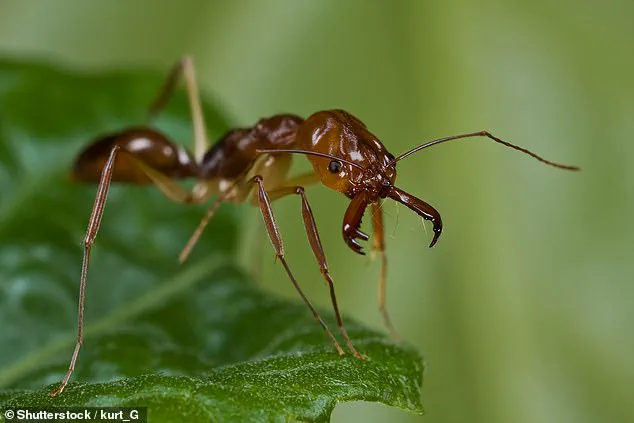
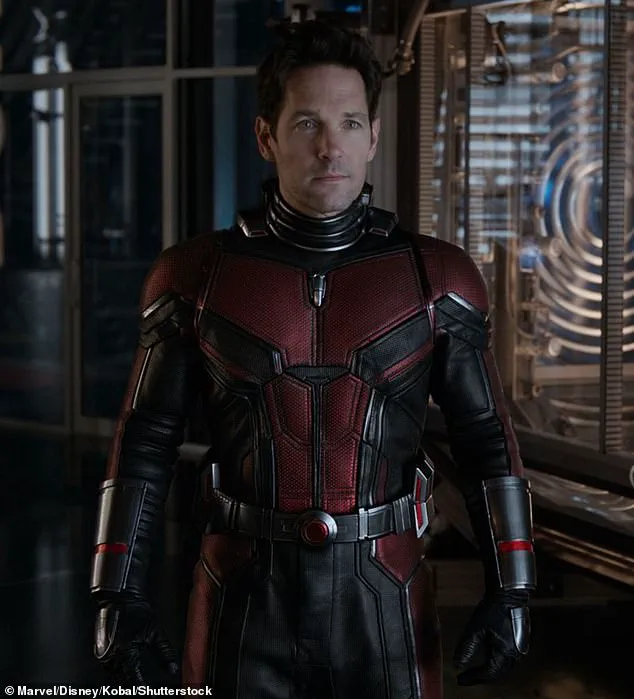
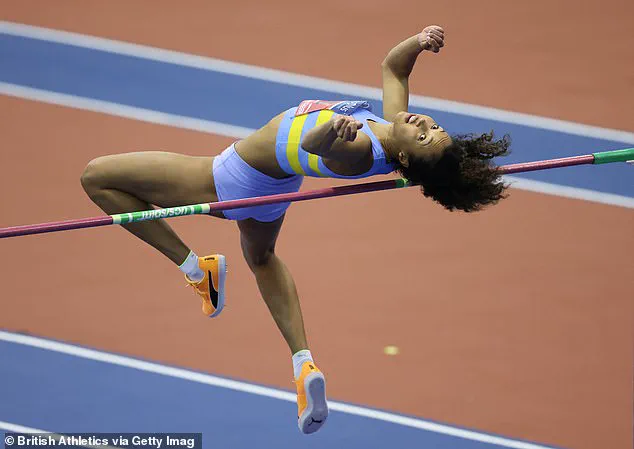
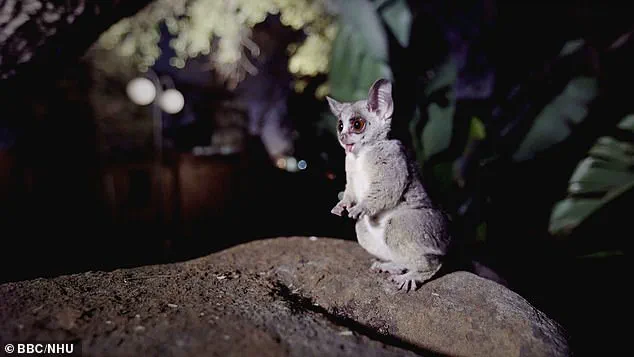
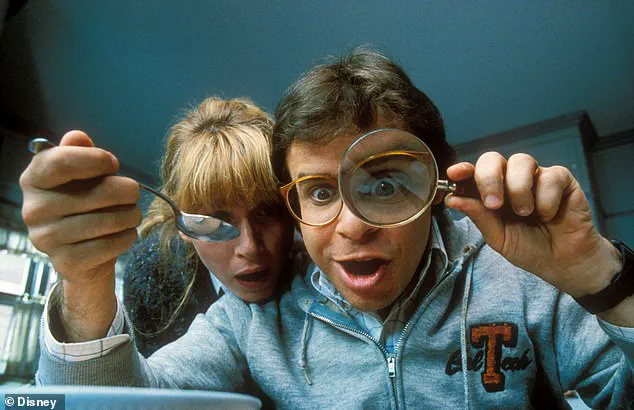
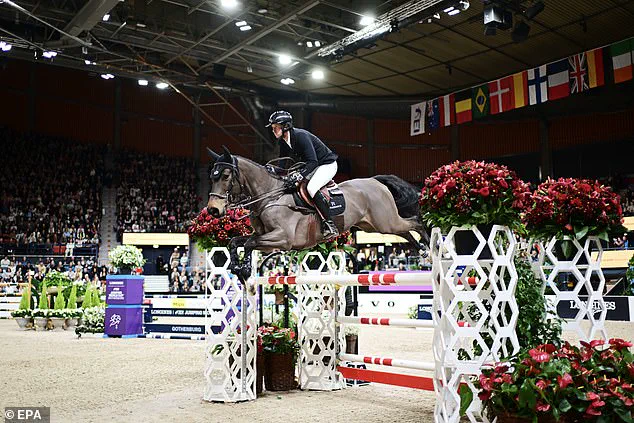
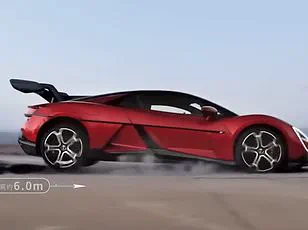
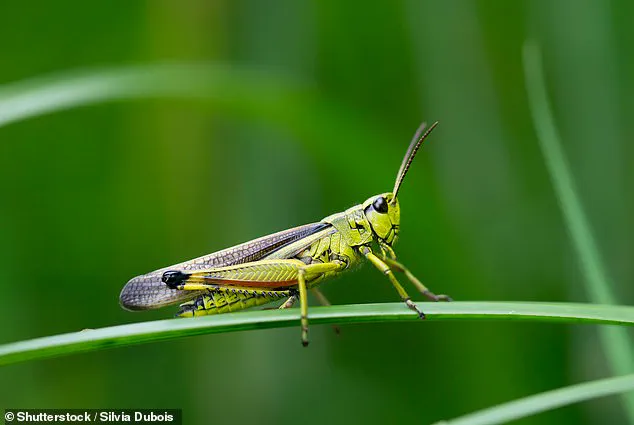